Sustainability in Beauty: An Innovative Proposing-Learning Model to Inspire Renewable Energy Education
Abstract
Renewable energy has become an important priority to the development of human society. The authors proposed an innovative “Proposing-Learning” model to improve the renewable energy education at the university level, in which the student community was extensively involved in the selection, development and assessment of a capstone project. In this project, a hybrid energy harvesting system prototype comprised of a bamboo wind turbine and solar panels was developed at the University of Vermont. The project idea was initially proposed through an online collective intelligence voting system, and then discussed in a committee comprised of students, faculty, staff and alumni members. A group of undergraduate students, representing different engineering disciplines, worked with the faculty advisors to create the prototype successfully. Good assessment was received from the students and local community for the project. Finally, the authors discussed the future effort to improve this education model and the potential applications of the hybrid renewable energy system.
Keywords: Sustainability, Renewable Energy, Education, Bamboo, Hybrid Energy System, Wind Turbine
Introduction
The utilization of renewable energy has become an important priority to the development of human society because it generates less greenhouse gas emissions than traditional energy sources (Jenning, 2009; Turner, 1999). The primary types of renewable energy include wind, solar, biofuels, geothermal and hydro power (Rosentrater and Al-Kalaani, 2006). It is reported that the installed capacities of renewable energy sources contribute 16.3% of U.S. total energy consumption by 2014 (Office of Energy Projects, 2014). Meanwhile, mid-term goals have been established for individual renewable energy sources. For example, the U.S. Department of Energy aims at supplying at least 20% of the nation’s electricity from wind power by 2030 (US DOE, 2008).
Technical features of the wind and solar energy infrastructure often enable them incorporated into the curriculums at schools to enhance the hands-on experience in renewable energy education, such as capstone design projects (Pecen and Nayir, 2010; Belu, 2011; Anderson et al., 2011). Despite these efforts, limited work exists to address two important aspects in renewable energy education. First, thoughts from students within the broader student community, not only the project members, could be better considered during the selection, development and assessment stages of the projects to extend the project’s societal relevance. Currently, the faculty members propose the projects, and the students join the project as team members to accomplish the tasks. Students outside the project team have little impact on the design goals of the proposed projects. Second, the inter-relationships between the energy infrastructure and the natural environment could be strengthened by developing more environmentally adaptable energy systems. For example, concerns are always expressed to wind turbine systems due to their intrusiveness to surrounding environment, such as their impacts on the landscape and the animals. Inclusion of natural materials in the design could also improve sustainable engineering to the natural world.
The objective of this paper is to implement an innovative “Proposing-Learning” model to improve the renewable energy education at the university level by addressing these concerns. In this work, a hybrid renewable energy system was developed using a bamboo wind turbine and solar panels at the University of Vermont. The paper is organized around the background of natural bamboo, the selection, development and assessment of the project.
Bamboo
Bamboo, comprised of hierarchical fiber and parenchyma structures, is a natural fiber-reinforced composite that evolves to resist wind loads (Fig. 1a). It is a group of perennial grasses in the grass family Poaceae, subfamily Bambusoideae, tribe Bambuseae (Liese, 1998). It grows in many tropical and temperature regions. The global bamboo forest is approximately 65,520 square kilometres (Lobovikov et al. 2007), 65% of which is in Asia. Bamboo is among the fastest-growing plants in the world (Farrelly, 1996), whose growth is based on the vegetative reproduction (Janssen, 2000; Banik, 1995). During this process, new rhizomes grow out of the ground to produce a secondary culm until the culm reaches a stable size (Chapman, 1996). Some large bamboo can reach the height of 15 to 30 m in about 2 to 4 months. An estimation classified bamboo into 75 genera and approximately 1500 species (Liese, 1998). Moso (Phyllostachys heterocycla pubescens) is the most widely distributed bamboo in the world (Liese, 1998).
The functionally graded bamboo structure has excellent mechanical properties, such as stiffness, strength and fracture resistance (Schulgasser, 1992; Tan et al., 2011). The bamboo culm includes approximately 40% cellulose fibers, 52% parenchyma tissue and 8% conduction tissue (Liese, 1998). Sclerenchyma fibers comprise the principal supporting tissues within the vascular bundles, whose primary chemical contents are cellulose, hemicellulose and lignin (Abdul Khalil, 2010). Surrounding the vascular bundles, there are parenchyma cells (Fig. 1b). In the cross section, the ratio of vascular bundles to parenchyma matrices decreases from the outside to the inside surface (Amada et al., 1997; Ghavami et al., 2003; Ray et al., 2004) (Fig. 1b). In the longitudinal direction, the narrowing of the bamboo results in a reduction of the parenchyma toward the culm head (Liese, 1998). Prior studies (Amada et al., 1997; Li, 2004; Ma et al., 2008; Nogata and Takahashi, 1995) showed that the elastic moduli and tensile strengths decrease from the outside to the inside surface, while the fracture resistance increases in the same direction (Tan et al., 2011). This is because less energy is invested to propagate cracks by splitting the fiber fences closer to the outside than breaking the lignin web closer to the inside surface, in which different bridging types and intensities were observed (Tan et al., 2011; Tan et al., 2014).

Fig. 1 (a) A Moso bamboo stalk in the field; (b) An optical image of functionally graded bamboo cross section. The dark areas are the fibers; while the light areas are the parenchyma matrices (Adapted from Tan et al., 2011)
Bamboo has been used by human beings over a long time for many applications. There are more than 1000 documented uses of bamboo (Bansal, 2002). In ancient China, bamboo books were crafted from culm strips strung on sting to record history (Loewe and Shaughnessy, 1999). Bamboo culms have also been successfully used to construct large scale structures, including buildings and bridges (Fig. 2). For example, Simon Velez built a bamboo church in Manizales, Columbia; a structure combining grace and sustainability (Fig. 2a). Bamboo is also a promising solution to build earthquake shelters in disasters (Richard, 2013; van der Lugt, 2008). A test bamboo house survived loads of 7.8 Kobe earthquake on a shake table (Vengala et al., 2008). The real proof occurred in 1991; approximately 20 bamboo houses survived near the epiccenter of 7.5 Richter scale earthquake in Costa Rica (Janssen 2000). Beside the utilization of bamboo culms, bamboo fibers could also be blended with polymeric matrices to produce high performance fiber-reinforced composites for many applications. As the ever-growing need for sustainable materials, bamboo fiber reinforced polymeric composites are widely used to manufacture various industrial products, such as racing bicycles (Fig. 2c), sport products and electric device packages (Tan, 2014). For example, more than 200,000 m2 plybamboo were used to build the roof at the Madrid-Barajas airport (Fig. 2d) (Lafarge, 2013). This award winning design project successfully synergized the sustainable materials and the daylight utilization aesthetically.

Fig. 2 (a) The bamboo church built by Simon Velez in Columbia (Adapted from Inspiration green, 2013); (b) The bamboo bridge in the crosswaters town at Guangdong, China (Adapted from EDSA, 2013); (c) A bamboo bicycle (Adapted from Boobicycles, 2013); and (d) The plybamboo roof at Madrid-Barajas airport (Adapted from Lafage, 2013).
Despite these studies, limited work exists to develop wind resistant bamboo structures even though bamboo is born to resist wind loads. Given its intrinsic to resist winds, bamboo could be a good material to produce high performance wind turbine blades (Brøndsted et al., 2005). Driven by this bio-inspired idea, a project was proposed to create a hybrid renewable energy system comprised of a bamboo wind turbine and solar panels, which are detailed in the following sections.
Methods
We implemented a “Proposing-Learning” model to improve renewable energy education for the students at the University of Vermont (UVM). The “Proposing-Learning” model denotes a collaborative effort among the student community, the faculty and the administrative staff in the selection, development and assessment stages of the proposed project.
The “Proposing-Learning” model synergizes the features of the “project-based” learning model and the “learner-centered education” model. Meanwhile, the uniqueness of the “Proposing-Learning” model is based on the active involvement of the students and instructors in the selection, development and assessment stages of the projects and creating substantial impact to a wider learner community.
- Project selection
In the beginning, a capstone project idea entitled “A hybrid energy harvesting system comprised of a bamboo wind turbine and solar panels” was submitted by a team consisting of faculty members and undergraduate students to the UVM Clean Energy Fund (CEF) committee. The CEF was created in response to students’ vision of UVM running efficiently on a fraction of its current energy needs, powered by clean, locally produced renewable energy that fulfills both the university’s needs and supports the local economy. Approved by UVM’s Board of Trustees in 2008, a $10 per semester student fee supports the fund, generating about $225,000 annually. A randomized survey of 419 students showed that 68 percent would pay $10 per semester for the development of clean energy on the UVM campus.
The CEF committee is comprised of student, faculty, administrative staff and alumni members at UVM. Each year, project ideas related to clean energy are collected over the campus, which are posted on-line via a crowdsourcing portal to receive votes from the UVM community to rank the ideas. After collecting the initial voting, the CEF committee members are fully involved in the review, discussion and selection of the proposed ideas.
In conventional capstone projects, communication only exists between two of the three sides in the projects, i.e., the faculty, students and administrators (Fig. 3). Information could not be shared among the three sides simultaneously. For example, the faculty and the administrators could come up with certain projects that may not be interesting enough to a wider student community. Or, the students may propose some projects to the administrators that are not be proper due to the technical or resource constraints. However, by forming the CEF committee, an extra dimension enables a synergized and direct communication among all of them. The ‘Proposing-Learning’ model substantially stimulates the interest and involvement of the student community and strengthens their learning motivations.

Fig. 3. A schematic of the “Proposing-Learning” model represented by the UVM Clean Energy Fund mechanism. The solid lines represent the two-way communication between two of the three sides, i.e., the faculty, the students and administrators. The dash lines represent the communication in the committee synergizing all three sides.
II. Project development
We proposed a hybrid renewable energy harvesting system comprised of a bamboo wind turbine and solar panels. The project included the design of the structural and the electrical modules. The project team consisted of two faculty members and four undergraduate students in the School of Engineering at UVM. Two students in mechanical engineering focused on the structural design; while the other two students in electrical engineering focused on the electrical system design. The development of the project is detailed in the following sections.
(1) General concept
The hybrid renewable energy system was comprised of a bamboo wind turbine and an array of solar panels. In the system, the energy harvested from the wind turbine and the solar panels were stored in the battery through an integrated control system (Fig. 4). The stored energy was then used to power LED lights installed on the center poles and the wind turbine blades. Beside ordinary illumination, we expected to see an aesthetically pleasing display of lights when the blades rotated. Moreover, the hybrid system was isolated from current power grids. To prove the concept, a prototype of the hybrid energy system was developed at the University of Vermont.
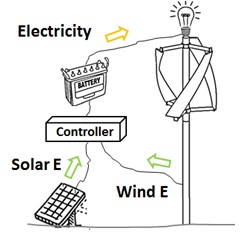
Fig. 4. A schematic of the hybrid renewable energy harvesting system consisting of a bamboo wind turbine and solar panels
(2) Structural Design
A vertical axis wind turbine (VAWT) was selected to harness the wind energy due to its good adaptability to building roofs in urban areas (Eriksson et al., 2008; Mertens, 2003). Initially, a literature review was performed to determine the design parameters of the VAWT systems (Johanson et al., 2014). The final selection was a Gorlov type wind turbine (Johanson et al., 2014) with helical blades. The design parameters are listed in Table 1, and the assembled wind turbine structure is shown in Fig. 5c.
Fabrication of the bamboo helical blades was essential to the project, which also directly affected the quality of wind turbine systems. We used a three-step strategy to manufacture the high quality helical bamboo wind turbine blades. Initially, 3D printing techniques (Lipson and Kurman, 2013) were implemented to produce inner blade skeletons using ABS polymers (Stratasys, Edina, MN). Accurate 3D geometrical models allowed precise control of the airfoils, the helical curves and the thickness. To reduce weight, arrays of circular cutouts were removed from the blade skeleton surfaces, and five struts were preserved to maintain the blade shape (Fig. 5a). Then, bamboo laminates were wrapped around the polymeric skeletons to form the blade surfaces. One-inch bamboo strips were attached to the skeletons from bottom to top using the PVA glue (Lineco Inc., Holyoke, MA). Prior layer surfaces were sand polished before the next layer was attached (Fig. 5b). Finally, the vacuum infusion method (Tan et al., 2013) was used to achieve the blade finish by placing the covered blade skeletons and EcoPoxy (Ecopoxy Inc., Canada) in vacuum for the final curing. Furthermore, 3D printed connectors were also created to link the blades and the vertical rotating shaft to horizontal bamboo frames. Beside wind turbine blades, structures were also designed for the electricity transfer module and the turbine-pole connection. To transfer electricity from the battery to the lights on rotating blades, a slip-ring system was developed using conducting brushes and metallic contacts. In addition, aluminum structural components were manufactured for both the center pole and the turbine-pole connection (Fig. 5c).

Fig. 5. Development of the bamboo wind turbine structure (a) a 3D printed polymeric skeleton for the helical wind turbine blades; (b) a fabricated bamboo laminated covered wind turbine blade skeleton; and (c) the assembled wind turbine structure on the roof.
Table 1. Design parameters for the vertical axis wind turbine system
Type | Height (m) | Diameter (m) | Aspect Ratio (H/D) | Pitch Angle (degrees) | Twist Angle (degrees) | Airfoil |
VAWT | 0.53 | 0.44 | 1.2 | 60 | 80 | NACA 0018* |
* Timmer, 2001
(3) Electrical System Design
The control system (Xia et al., 2003, Franco et al., 2004) was implemented using a microcontroller to perform multiple functions, which included regulating the battery charge and voltage supply, monitoring the motor and solar panels and controlling the LED light patterns. Meanwhile, it also controlled a driver circuit to provide a start-up driving force to start the motor rotation by overcoming the initial large torque of motor device.
For the electrical aspect, the system must be able to effectively harvest energy so as to generate sufficient voltage from the sunlight and the wind for the battery charging, which is responsible to power on a set of LED lights. For safety consideration, it is important to protect the battery from overcharging or over draining, and to control and switch power source for the battery charging between the solar panel and the wind turbine. The proposed control circuit design (Xia et al., 2007, 2008) is shown in Fig. 6a. The output voltages generated from the motor and the solar panel were treated as analog input signals to the microcontroller. The microcontroller was programmed to connect either wind turbine motor or solar panel output signal to the battery for power charging while leaves the other one open. The battery terminal voltage level was sampled and monitored by an analog to digital converter (ADC) circuit inside the microcontroller, which protects the battery from being overcharged or over drained. Once the voltage level is monitored beyond or below the preset threshold values, control circuit will act to disconnect the battery from the power source or the LED lights. The proposed circuit had been designed and tested. Fig. 6a shows the layout of the printed circuit board (PCB), and Fig. 6b illustrates the fabricated circuit board with circuit components populated. The PCB was a two sided, high performance board, including plated through-holes, a top layer silkscreen, and a solder mask to prevent trace oxidation. Banana connectors were placed on each power node to prevent shorts between sources and ground so as to achieve high reliability. LED lights were connected via standard receptacle connectors through single row header pins and controlled by the microcontroller, which switch the lights on or off states between nighttime and the daytime.
III. Project assessment
The project team was assessed throughout a two semester capstone design course with deliverables, design reports and design presentations. A demonstration of the prototype and the results of device testing were also required. A poster and video were authored and presented at the Design Night, an event that the local residents in Burlington areas could attend. Lastly, three exams were used to determine the learning of each student team member related to the project performance. Exams, deliverables and design reports were graded by the course instructors and design presentation evaluated by peers, industry members and faculty.
Results
- Project selection
The selection of the hybrid project included both the online review and the committee panel discussions, during which the UVM students were extensively involved in. In the online review, ideas on renewable energy were collected and shared within the UVM community. Each person with a UVM email address could log into the Clean Energy Fund system and vote for the favorable ideas. The voting numbers were collected on both the numbers of thumbs up (“like”) and thumbs down (“dislike”). The net votes were calculated by subtracting the “dislike” from the “like” numbers. If the “dislike” numbers exceed the “like” numbers, a negative number appears for the net votes. In real-time, UVM campus members could see posted ideas elevating up or down based on net votes.
A quantitative analysis was performed to evaluate the results in the online review stage. There were 38 ideas submitted to the UVM Clean Energy Fund committee in 2012-13. The indices were defined as follows. Total votes were the numbers consisting of all the “like” and “dislike” votes. Supporting rates were the ratios between the “like” and the total votes. The average net cost was the ratio between the proposed budget dollars and the net votes, representing the cost-effectiveness of the proposal. Because some proposals did not include a budget number, a zero value was assigned to the average net cost. The hybrid energy system was the NO. 5 proposed idea in the array. In Figs. 7a and 7b, the data showed that the NO.5 proposal won substantial popularity (total votes) and the supporting rates. In Fig.7c, it showed that average net cost of the hybrid project was close to the low end in the proposed ideas.
Before the panel discussion, the hybrid team was invited to present the proposed idea to the committee for potential questions. After that, several committee meetings were organized to discuss the proposed ideas based on the innovation, the budget plan, the project team, and the results collected from online review stage. Finally, the award decision was notified to the hybrid team. Students, not only the project members, but also the committee members and the voters, actively participated in the entire selection process. Both vote results and the final decision exhibited that this ”Proposing-Learning” mechanism was effective in selecting projects synergizing the interests of the student community, the idea innovation and budget plans.
II. Project development
A prototype of the hybrid energy system was developed at the University of Vermont during the school year of 2013-2014. In this period, group meetings were scheduled every other week to discuss the project progress, attended by the two faculty members, the four team students and an administrator staff. Technical details were revisited and discussed between the faculty advisors and the student members. Effective communication was established between the structural and the electrical groups to coordinate the needs from both sides. The next-step tasks were also elucidated for each group member for the following meeting. The administrative staff from the Clean Energy Fund committee provided essential help on the logistics, such the roof access authorization for the system installation.
After the group meetings, the progress was periodically briefed to the committee members, during which feedback and suggestions were collected and transferred back to the hybrid team. The student team members were also required to present the project status to broader audience based on the senior capstone project schedules, including the literature review, the system development, the laboratory tests and the field demonstration.
The hybrid energy system was tested on the Votey building roof at UVM. Two groups of snapshots are shown to illustrate the field performance of the hybrid energy system at both day and night times (Figs. 8a-8f). The background was the Ira Allen Chapel at UVM. When the hybrid system was installed in the day time (Figs. 8a-8c), we noticed that a good compatibility existed between the bamboo wind turbine and the surrounding environment. Due to the inherent connections between bamboo plants and the earth, the wind turbine could be treated more like a giant plant than an artificial structure. During the night, the stationary lights on the center pole provided enough illumination for the adjacent area, while the rotating lights on the blades displayed aesthetically pleasing patterns.
In the end, the hybrid energy system was demonstrated in the ‘Design Night’ event organized by the College of Engineering and Mathematical Sciences of UVM (Fig. 9). Local residents, ranging from kids to seniors in the Burlington area, were invited to attend the event and share their excitement of the innovative capstone projects. In the event, the hybrid energy system was warmly embraced by the UVM community, attracting a lot of attention and interests from the event attendees. Suggestions were collected from students, parents and local industries for the future development of the project. The hybrid team also received many invitations from the local schools in Burlington areas to demonstrate the project to more K-12 students in the future.

Snapshots of hybrid energy system in (a-c) the day time, (d-f) the night time; the background is the Ira Allen Chapel at the University of Vermont. (Fig. 8 (f))

Fig. 9. A picture of the demonstration of the hybrid energy system in the Design Night event at UVM in 2014.
III. Project assessment
The evaluation of the final presentation was a good indication of the students’ performance in the proposed project. They showed the input of student peers, faculty and industry members who were all present at the presentations. The student team was assessed on Understanding, Preparedness, Enthusiasm, Comprehension and Content using a four point scale (Table. 2). For Understanding, the team was found to show a full and thorough understanding of all aspects of the project. Similarly, their Comprehension score showed the ability to accurately answer all of the audience questions posed related to the design, analyses and testing. The Content score indicated the completion of the project to their client’s satisfaction. Lastly, Preparedness and Enthusiasm ratings indicated that they were well prepared for their presentation and generated strong interest in their work with their presentation style. Overall, their final presentation grade was in the top five percent as were all their oral presentation work. A difference can be found between the hybrid system project and other capstone projects proposed in conventional ways.
As with most teams, there are strengths and weaknesses. This team was not as strong in written design reports as they were in oral presentation. There is a need to provide more instruction, peer and practitioner-review of written presentations for engineering students in the overall course.
The project has also attracted attention from different public medias, such as UVM communications (Brown, 2014), www.designnews.com (Montalbano, 2014), www.greenenergytimes.net, www.energymatters.com.au, www.energyharvestingjournal.com, www.openideo.com, www.lifewithbamboo.com/ etc. A video named “UVM Hybrid Energy Harvester” is uploaded to www.youtube.com to illustrate the working condition of the system.
Discussion
A prototype of the hybrid renewable energy system, consisting of a bamboo wind turbine and solar panels, was developed by a team comprised of faculty members and undergraduate students at UVM. In this project, an innovative “Proposing-Learning” model was implemented to enhance the involvement of students to every stage of the project, including the selection, development and the assessment stages. In the selection stage, the online review forum effectively promoted the interests from the student community in renewable energy topics. In the development stage, a group of undergraduate students worked closely with the faculty advisors to develop an innovative renewable energy system comprised of a bamboo wind turbine and solar panels. In the assessment stage, peer-reviewed feedbacks exhibited the effectiveness of this model mechanism in enhancing the renewable energy education. By using this model, we could promote the renewable energy education by making an impact to the student community before, during and after the project. In the future, we will improve this “Proposing-Learning” model by strengthening the post-project assessment. We plan to collect online comments and ratings for the completed projects from the student community. We will also organize seminars on-campus to share the experience with the students by creating direct communication within the UVM community. Through these activities, we expect to collect suggestions and new ideas for the future development of these projects and this education model.
Meanwhile, we plan to develop more environmentally compatible energy infrastructure based on the developed prototype of the hybrid energy system. First, due to the intrinsic to resist wind loads, bamboo laminates are excellent solutions to manufacture small and middle scale distributed wind turbine blades. Second, in the field demonstration, bamboo wind turbines are less intrusive to the surrounding environment because of their inherent connection to earth. The idea of “planting” wind turbines will make the renewable energy utilization more appealing and environmentally adaptable. A more sustainable relationship will be established between the energy infrastructure and the landscape of mountains, farms and ocean shorelines. Third, the proposed hybrid system is isolated from current power grids, making it a proper option for remote areas. Due to the abundance of bamboo plantation, the bamboo wind turbines could be developed and used in many parts of the world, such as developing and underdeveloped regions. The renewable energy utilization in these areas will make a significant impact on the sustainable development of the local communities. Finally, the light patterns on the rotating blades could be tailored to meet various needs, such as the warning, emergency, advertising and entertainment. In the future, we will conduct a series of experiments to quantitatively characterize the long-term performance of the hybrid energy system.
Conclusions
We implemented a ”Proposing-Learning” model to inspire renewable energy education at the University of Vermont by developing a hybrid energy system comprised of a bamboo wind turbine and solar panels. Born to resist wind loads, bamboo is featured by the hierarchical structures of fibers and parenchyma cells, making it remarkable in strength, stiffness and fracture resistance. Despite many applications in housing and industrial products, limited research exists to develop bamboo wind turbines. By using the “Proposing-Learning” model, we extensively involved the students in the selection, development and assessment stages of the hybrid energy project. The results showed that the model effectively improved the renewable energy education at the university level. Meanwhile, an innovative renewable energy system synergizing sustainability and beauty was developed during the project period. In the future, we will strengthen the post-project assessment, and further develop the hybrid renewable energy system to meet various needs.
Acknowledgements
The authors greatly appreciate the funding from the UVM Clean Energy Fund to support the project. We are also grateful to the help from the Senior Experience in Engineering Design program at the School of Engineering, and the 3D printing support from the College of Engineering and Mathematical Sciences at UVM. Meanwhile, we very much thank the Office of Undergraduate Research at UVM for providing the summer support for students in this project.
Table 2. A summary of the peer review table for final project presentations.
CATEGORY | Points: 4 | 3 | 2 | 1 | Hybrid Team Score | Overall Team Averages |
Understanding | Presenters show a full and thorough understanding of the topic. | Presenters show a good understanding of the topic. | Presenters show a good understanding of certain parts of the topic. | Presenters do not seem to understand the topic very well. | 4.00 | 3.60 |
Preparedness | Presenters are completely prepared and have obviously practiced. | Presenters seem pretty prepared but might have needed some more practice. | Presenters are somewhat prepared, but it is clear that more practice was needed. | Presenters do not seem at all prepared or ready to present. | 3.90 | 3.85 |
Enthusiasm | Facial expressions and body language generate a strong interest and enthusiasm about the topic in others. | Facial expressions and body language sometimes generate a strong interest and enthusiasm about the topic in others. | Facial expressions and body language are used to try to generate enthusiasm, but seem somewhat faked. | Very little use of facial expressions or body language. Did not generate much interest in topic being presented. | 3.80 | 3.40 |
Comprehension | Presenters are able to accurately answer almost all questions posed by the audience about the topic. | Presenters are able to accurately answer most questions posed by the audience about the topic. | Presenters are able to accurately answer a few questions posed by the audience about the topic. | Presenters are unable to accurately answer questions posed by the audience about the topic. | 4.00 | 3.60 |
Content | Presenters provide a Problem Statement, Description of design being built, Testing to be performed and Open issues | Presenters did not provide one (1) of the previous list of seven (4). | Presenters did not provide two (2) of the previous list of four (4). | Presenters did not provide three (3) or more of the previous list of four (4). | 4.00 | 3.80 |
Total Scores | 19.80 | 18.30 |
Reference
Abdul Khalil, H., Bhat, I., Jawaid, M., Zaidon, A., Hermawan, D., Hadi, Y. Bamboo fibre reinforced biocomposites: A review. Materials and Design, (42)353-68, 2012.
Amada, S., Ichikawa, Y., Munekata, T., Nagase, Y., Shimizu, H. Fiber texture and mechanical graded structure of bamboo. Composites Part B: Engineering, 28(1):13-20, 1997.
Anderson, J., Hughes, B., Johnson, C., Stelzenmuller, N., Sutanto, L., Taylor, B. Capstone project report: design and manufacture of a cross-flow helical tidal turbine, University of Washington, 2011.
Banik, R. L., A manual for vegetative propagation of bamboos, Technical Report-International Network for Bamboo and Rattan, Beijing, China, 1995.
Bansal, A. K., Zoolagud, S. Bamboo composites: Material of the future. Journal of Bamboo and Rattan, 1(2):119-30, 2002.
Belu, R. G. Renewable energy based capstone senior design projects for an undergraduate engineering technology curriculum. American Society for Engineering Education, 2011.
Brøndsted, P., Lilholt, H., Lystrup, A. Composite materials for wind power turbine blades. Annual Review Material Research, 35:505-38, 2005.
Boobicycles, Boo fixie, Available at: http://boobicycles.com/gallery/?id=72157627024205218&title=Boo%20Fixie [accessed December 25th, 2013].
Brown, J. UVM engineers build tiny renewable wind turbine for developing world, 2014; Available at: http://www.uvm.edu/~uvmpr/?Page=news&storyID=19600.
Chapman, G. P., The biology of grasses, CABI Press, Wallingford, United Kingdom, 1996.
EDSA, Crosswaters ecolodge. Available at: http://www.edsaplan.com/en/node/651 [accessed December 23rd, 2013].
Farrelly, D., The book of bamboo: A comprehensive guide to this remarkable plant, its uses, and its history, Sierra Club Books Press, San Francisco, CA, United States, 1996.
Franco, S., Song, P., Xia, T., and Weger. A. J. Broken scan chain diagnostics based on time-integrated and time-dependent emission measurements. In Proceedings of International Symposium for Testing and Failure Analysis, pp. 52-57. 2004.Ghavami, K., Rodrigues, C., Paciornik, S. Bamboo: Functionally graded composite material. Asian Journal of Civil Engineering (Building and Housing), 4(1):1-10, 2003.
Inspiration Green, Bamboo buildings: Grow it, build with it, 2013; Available at: http://www.inspirationgreen.com/bamboo-buildings.html, accessed at 06/2014.
Janssen, J. J., Designing and building with bamboo, Technical Report-International Network for Bamboo and Rattan, Beijing, China, 2000.
Jennings, P. New directions in renewable energy education. Renewable Energy, 34(2):435-39, 2009.
Karl Johanson, Justin Dao, Zachary Basch, Hunter O’ Folan, Wind and solar powered stree lights,
the Univeristy of Vermont, 2014.
Lafarge, Barajas airport, a window on Madrid, Available at: http://www.lafarge.com/contribute-better-cities/our-completed-projects/barajas-airport-window-madrid, [accessed September 13, 2014].
Li, X. B. Physical, chemical, and mechanical properties of bamboo and its utilization potential for fiberboard manufacturing. Master thesis, Louisiana State University, 2004.
Liese, W. The anatomy of bamboo culms. Beijing, China: Technical Report-International Network for Bamboo and Rattan; 1998.
Lipson, H., Kurman, M. Fabricated: The new world of 3d printing. John Wiley & Sons; 2013.
Lobovikov, M., Ball, L., Guardia, M., Russo, L. World bamboo resources: A thematic study prepared in the framework of the global forest resources assessment 2005. Rome, Italy: Food and Agriculture Organization of the United Nations Press; 2007.
Loewe, M. and Shaughnessy, E. L., The Cambridge history of ancient china: From the origins of civilization to 221 BC, Cambridge University Press, Cambridge, United Kingdom, 1999.
Ma, J. F., Chen, W. Y., Zhao, L., Zhao, D. H. Elastic buckling of bionic cylindrical shells based on bamboo. journal of Bionic Engineering, 5(3):231-38, 2008.
Montalbano, E. Video: bamboo-based hybrid wind turbine aimed at developing world, 2014; Available at: http://www.designnews.com/author.asp?section_id=1386&doc_id=275716.
Nogata, F., Takahashi, H. Intelligent functionally graded material: Bamboo. Composites Engineering, 5(7):743-51, 1995.
Office of Energy Projects, Energy Infrastructure Update, 2014.
Ray, A. K., Das, S. K., Mondal, S., Ramachandrarao, P. Microstructural characterization of bamboo. Journal of materials science, 39(3):1055-60, 2004.
Shen, S., The art of survival: Case study of crosswaters ecolodge, Nankunshan, Guangdong, Urban Space Design, 3:64-73, 2007 (in Chinese).
Turner, J. A. A realizable renewable energy future. Science, 285(5428):687-89, 1999.
Pecen, R., Nayir, A. Promoting stem to young students by renewable energy applications. Modern Electric Power Systems, 2010 Proceedings of the International Symposium, 2010.
Richard, M. J. Assessing the performance of bamboo structural components. PhD Thesis, University of Pittsburgh, 2013.
Rosentrater, K. A., Al-Kalaani, Y. Renewable energy alternatives-a growing opportunity for engineering and technology education. The Technology Interface, 5(1):1-12, 2006.
Schulgasser, K., Witztum, A. On the strength, stiffness and stability of tubular plant stems and leaves. Journal of theoretical biology, 155(4):497-515, 1992.
Timmer, W. Two-dimensional low-Reynolds number wind tunnel results for airfoil NACA 0018. Wind engineering, 32(6):525-37, 2008.
Tan, T., Rahbar, N., Allameh, S., Kwofie, S., Dissmore, D., Ghavami, K., Soboyejo, W. Mechanical properties of functionally graded hierarchical bamboo structures. Acta biomaterialia, 7(10):3796-803, 2011.
Tan, T., Ren, F., Wang, J. J.-A., Lara-Curzio, E., Agastra, P., Mandell, J., Bertelsen, W. D., Lafrance, C. M. Investigating fracture behavior of polymer and polymeric composite materials using spiral notch torsion test. Engineering Fracture Mechanics, 101:109-28, 2013.
Tan, T. Bamboo inspired materials. Bio-inspired design, Cambridge University Press, Cambridge, United Kingdom, 2014 (In press).
Tan, T., Tian, X., O’Folan, H., Dao J., Basch, Z, Johanson, K., Ozeki, M., Smith, M. Sustainability in beauty: a review and extension of bamboo inspired materials. Proceedings of 13th International Symposium on Multiscale, Multifunctional and Functionally Graded Materials, October, 2014, SP, Brazil, 18-21.
US Department of Energy, 20% wind energy by 2030: Increasing wind energy’s contribution to U.S. Electricity supply. DOE/GO-102008-2567, Energy Efficiency and Renewable Energy, 2008.
Van Der Lugt, P. Design interventions for stimulating bamboo commercialization – dutch design meets bamboo as a replicable model. PhD Thesis, Delft University of Technology, 2008.
Vengala, J., Jagadeesh, H. N. and Pandey, C. N., Development of bamboo structures in India. Modern bamboo structures, Taylor & Francis Press, London, United Kingdom, 51-63, 2008.
Xia, T., Lo, J.C. On-chip short-time interval measurement for high-speed signal timing characterization. In Proceedings of IEEE Asian Test Symposium, 2003.
Xia, T., and S. Wyatt. “High Output Resistance and Wide Swing Voltage Charge Pump Circuit.” In Proceedings of the 9th international symposium on Quality Electronic Design, pp. 114-117. IEEE Computer Society, 2008.
Xia, T., and H. Zheng. “Timing jitter characterization for mixed-signal production test using the interpolation algorithm.” Industrial Electronics, IEEE Transactions on 54, no. 2 (2007): 1014-1023.